The grand aim for Work Package 5 is to provide the underpinning knowledge and modelling approaches required to enable new engine developments that are reliant on coolant delivery and thermal management.
Thermal management is critical to deliver new engines that will enable net zero. Whether the new engines utilise new fuels or are hybrid or fully electric they will require lubrication and cooling at higher heat loads than previous engine architectures. This requires fundamental data to enable robust modelling techniques that will ensure new designs can make this step change in thermal management need. The reliable prediction of heat generation, transport and cooling is key to modelling the operating thermal environment and thermal-mechanical response of the machine structures.
Work Package 5 in Cornerstone will tackle these issues by:
- Creating test facilities to capture experimental data for coolant droplet vaporisation and breakup, providing the underpinning data and correlations for modelling these key aspects of coolant motion in a hot highly sheared two phase flow environment. This is undertaken under task 5.1.
- Further develop both test facilities and techniques to capture shear driven film flow behaviour, particularly the interface turbulence characteristics, providing fundamental data enabling model development and validation. This is undertaken under task 5.2.
- Develop a new test facility to capture data on the oil behaviour and mechanisms of heat generation under highly loaded contacts to provide validation data to both a Thermo-Elastro-HydroDynamics and CFD model of the situation. This is undertaken under task 5.3.
- Capture high temperature material properties for Ti-6Al-4V material with high strength to weight ratio, utilising 3D additive manufacturing to produce samples, and provide recommendations for Finite Element Modelling of this material. This is undertaken under task 5.4.
Task 5.1
Oil supplied for lubrication and cooling of surfaces is often atomised and interacts with the surrounding air flow before deposition on surfaces. This process significantly affects the heat transfer between the oil film and wall, as well as oil chemistry and degradation. It is therefore essential to understand the lubricant droplet vaporisation and breakup dynamics in a convective heating environment in order to adequately model the subsequent surface cooling.
The standard testing for evaporation rates are performed in heated crucibles, where the heating rate and surrounding gases are controlled. Whilst this provides a consistent data set for comparison of behaviour of different fluids under the same conditions those conditions do not match those experience by the oil in an engine. This project therefore is generating data on evaporation behaviour in conditions that better represent the phenomena that would be present in an engine.
The rig (figure 1 and 2) provides heated air, conditioned by passing through a settling chamber with a section of honeycomb and meshes in the flow passage, which is discharged through a nozzle. A droplet is suspended in this airflow with the temperature of that droplet being monitored continuously. The droplet shape is captured by high speed video that is then interrogated to analyse the change in morphology of that droplet over time in the hot air stream. The temperature and flow rate of the airflow is controlled and variable. By studying the morphology of the droplet the rate of evaporation can be established.

5.1 Experimental rig
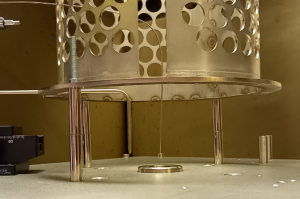
5.1 Experimental rig close up
This work is being led by David Hann https://www.nottingham.ac.uk/engineering/people/david.hann
Task 5.2
Turbulence generated by gas shear on a liquid free-surface plays an important role in the momentum transfer within multiphase thermal management. Accurately modelling these interface turbulence effects is essential to the reliable prediction of component power losses, lubricant distribution and heat transfer. Past research has demonstrated the high sensitivity of the state-of-the-art CFD simulation methods to a choice of the turbulence damping parameter, a parameter within the relevant sub-model. This sensitivity represents a source of uncertainty in the application of CFD to sheared liquid films, which impacts the reliability and resilience of prediction methods.
Currently, there is a limited understanding of the nature of this interface turbulence damping and how to best represent it in computational models. Available modelling techniques focus on using a rigid wall analogy of the liquid interface turbulence damping where turbulence dissipation close to the liquid interface is assumed to be equivalent to that near a rigid wall. The turbulence damping factors are often tuned to match observed flow characteristics from experiments and are grid independent. Similar approaches have been presented where the tuneable damping factor is removed and source terms are introduced at the interface to ensure that the air flow turbulence is completely dissipated close to the interface and turbulence viscosity is zero in the vicinity of the interface.
This limited understanding has led to two separate aspects of fundamental research within this work package; the first is an experimental investigation of simultaneous measurements in both the gas and liquid and the second is to look at an alternative modelling approach.
The experimental approaches include simultaneous PIV (particle image velocimetry), BBLIF (brightness based laser induced fluorescence) as well as the use of film height and pressure sensors to capture both point and full field measurements of the gas and liquid behaviours across a wide range of liquid and gas flow rates. The gas and liquid are co-flowing, with the liquid film being pumped into the 2m long test section before the shearing gas flow is introduced over the top of the film.
This work is being led by David Hann https://www.nottingham.ac.uk/engineering/people/david.hann
The modelling approach uses machine learning to use both high order modelling and experimental data to produce a low order RANS CFD model that can be used by industry to produce resilient and reliable simulations.
This work is being led by Richard Jefferson-Loveday
https://www.nottingham.ac.uk/engineering/people/richard.jefferson-loveday
Covid19 had a significant impact in 2020 with university buildings being closed as part of the national lockdown from late March, the first buildings re-opening in July and the final facilities and buildings required for Cornerstone opening in late September 2020. Throughout 2021, although there were national lockdowns our experimental facilities remained opened. Most staff were working from home between January – June 2021 unless they were required to be on site. The pandemic has continued to impact work throughout the whole of 2021, social distancing and hygiene requirements in the first six months caused tasks to take longer in the laboratories and there has been an ongoing impact relating to the supply of some components and consumables due to the global shortage of cpu/chips and raw materials. All of our staff have gone above and beyond to keep us operational and meeting milestones and deliverables although there has been some inevitable impact causing slippage on some milestones/deliverables.